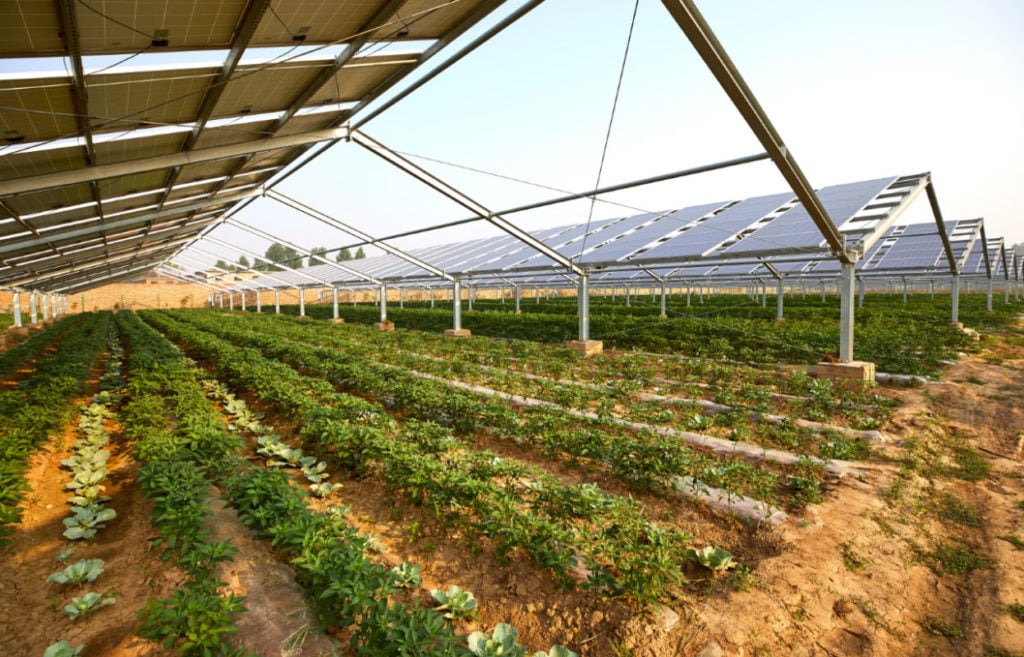
The optimal combination of PV and agricultural production in agrivoltaic systems is the subject of extensive scientific exploration. Hugo Sánchez Ortiz report reports on some of the findings of research into how best to balance land use for energy and food production.
The climate emergency drives the need for a transition to a carbon-neutral society. At the same time, it is essential to balance sustainability goals with the demand for vital services such as energy and food security. Renewable energies, particularly solar photovoltaics (PV), have taken centre stage in this transition. Thanks to its modularity and the historical reduction of its levelised cost of electricity (LCOE), PV has emerged as a leading technology in the energy transition. However, PV faces practical limitations, such as temporal mismatch between energy generation and demand, and the extensive land use it requires, which increasingly competes with other land-based activities, such as agriculture.
Unlock unlimited access for 12 whole months of distinctive global analysis
Photovoltaics International is now included.
- Regular insight and analysis of the industry’s biggest developments
- In-depth interviews with the industry’s leading figures
- Unlimited digital access to the PV Tech Power journal catalogue
- Unlimited digital access to the Photovoltaics International journal catalogue
- Access to more than 1,000 technical papers
- Discounts on Solar Media’s portfolio of events, in-person and virtual
Or continue reading this article for free
The theoretical energy rate conversion – approximately 22% for commercial panels – necessitates relatively large tracts of land for PV systems. Expanding installed capacity as we are currently doing may eventually create conflicts with other land uses, such as farming. To address this, Goetzberger and Zastrow [1] propose a simple but powerful idea in 1982: to combine agricultural and energy generation activities on the same land.
This concept, called agrivoltaics (APV), is based on the understanding that both food and energy production (using PV) rely on the same resource: sunlight. Would it not make sense to harmonise these activities to avoid competition over the land? With this idea, agrivoltaics was born, though it saw little development for over 30 years.
AgriPV in theory
Before examining the current status of APV, let’s consider how energy and food production make use of the solar resources. It is well known that sunlight provides energy, carried by energy particles called photons, that can excite specific semiconductor structures, realising electrons and, with the movement of these electrons, generate electricity. The process is called photoelectric effect. In this effect, without considering the limits and constraints of the materials, the larger the photon energy intensity the larger photo-generated current can occur. This process has been well used in the solar PV industry.
Growing food utilises the same sunlight but in a different way. While the photoelectric process exploits the electromagnetic properties of the semiconductor, photosynthesis is based on an organic chemistry process through which molecular changes produce energy. With particular variations between crops, the plants convert carbon dioxide (CO2) into carbohydrates, storing these carbohydrates in the food.
This process is not only dependent on sunlight, but requires additional elements such as water, nitrogen and phosphorus among others, creating a more complex and not linear process than the photoelectric effect. Variability in sunlight and other factors affects photosynthetic productivity, creating a saturation curve that shows diminishing returns in light intensity.
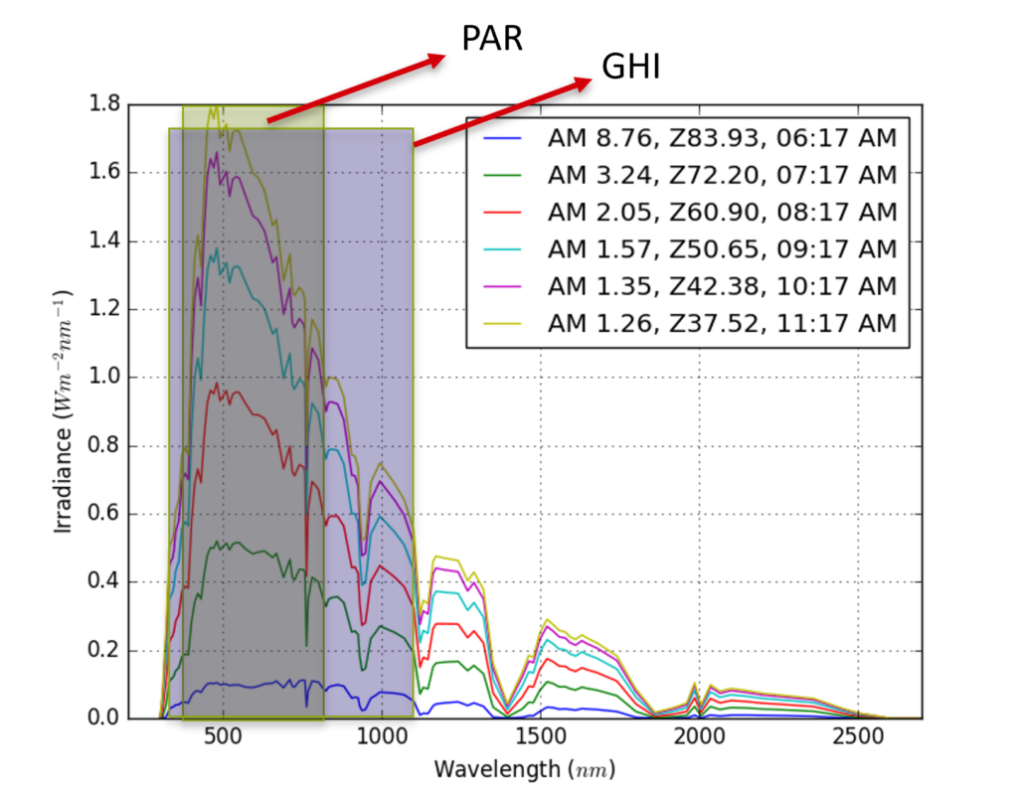
At this point, it is important to clarify some differences regarding the solar spectrum portions used in each process. Unfortunately, we are not able to take advantage of all the valuable resource of the Sun, due to physical and biological limitations; however, day after day, scientific and technological advances are pushing these boundaries. The solar spectrum can be divided into three sections: ultraviolet (around 5% of what we receive), visible light (approximately 42%) and infrared (53%). Thus, only around half of the solar spectrum is usable.
Nevertheless, despite these limitations, we primarily make use of the highly energetic portion of the Sun. Now, the spectral needs of PV and crops differ: silicon PV uses the range of the spectrum from 400nm to 1,100nm, in what is termed Photovoltaic Active Radiation (PVAR); meanwhile, crops utilise the spectra from 400-700nm, in what is defined as Photosynthetically Active Radiation (PAR). Even though these ranges overlap significantly, they are distinct, and it becomes clear that optimising synergy between the two systems is essential.
In summary, PV modules require as much sunlight exposure as possible, while crops can reach a saturation point. After this point, the crops can experience problems that even can damage the plant (as anyone who has seen sun-scorched plants with inadequate water knows). Therefore, if one component (PV) requires as much sun exposure as possible and the second component (crops) requires more regulated sun exposure, does it not make sense to combine both food and energy production on the same land? Maybe it would be possible to maximise the resources taking advantage of this index? And the potential of resource optimisation is one of the main motivations driving researchers, industry and decision makers into push the development of agrivoltaics.
Agrivoltaics in practice
Although the idea of agriPV was first established in the 1980s, it was not until the latter half of the 2010s that agrivoltaics becomes a serious topic of research and project development focus. This delay was due to the historical decrease in the price of PV, the urgency for a clean energy transition and some grid saturation problems.
First, the variability of solar energy makes it challenging to match the generated electricity with demand, which in turn limits the selection of suitable areas for new projects. Second, the available spaces near to demand are occupied by other activities such as farming. This leads to a land use conflict that is becoming more common in the planning process for new projects. This suggests that it is necessary to look for optimised alternatives to balance the limited resources.
System optimisation has been one of the centres of focus for researchers and project developers. As the field is relatively young, there are no rules set in stone about what are the optimal configurations to implement for these systems, considering the variation of the crops and the need to adjust to local food production and farming dynamics. All this represents a huge potential field of research, and researchers around the world are indeed already exploring different configurations and variations with some promising preliminary results.
In the case of Germany, the German Institute for Standardization [2] (DIN from Deutsches Institut für Normung) proposes two categories for the implementation of APV and sets requirements for these systems. Category I encompasses installations where farming activities are conducted under the PV modules. Category II, meanwhile, includes installation set-ups in which farming activities are developed between the PV system rows.
Both categories classify different crops according to their particular characteristics: A) permanent and perennial crops; B) annual and multi-year crops; C) permanent grassland for mowing; and D) permanent grassland used as pasture. The classification also considers three main mounting systems: 1) elevated tilt angle; 2) vertical bifacial systems; and 3) solar trackers.
Based on this classification, a recent study developed by our team evaluates the performance for the systems proposed in the DIN SPEC 91434, examining tilt elevated angle (with crops between and behind), a vertical bifacial east-west system and tracker systems [3]. One of the key findings was the fact the modifications in the system topology does not affect the performance or specific energy yield (just about 10% between a PV optimised case versus the vertical bifacial east-west configuration). Do not get confused about this term: ‘specific energy yield’ refers to the energy generated per kilowatt peak installed, so while fewer modules on a field mean less total production, the per-unit performance remains largely unaffected. This metric, however, does impact the revenue of an installed system.
On the other hand, the system topology plays a critical role in the overall performance of the system. According to the distribution of the modules on the field, the remaining light – i.e. the amount of light that will be received by the crops – will change. In our study, we found that could change up to 52% for the case of vertical bifacial east-west. In this case, the allocation of the light needs to be analysed according to the crops, because the crops have several shade-tolerant behaviours.
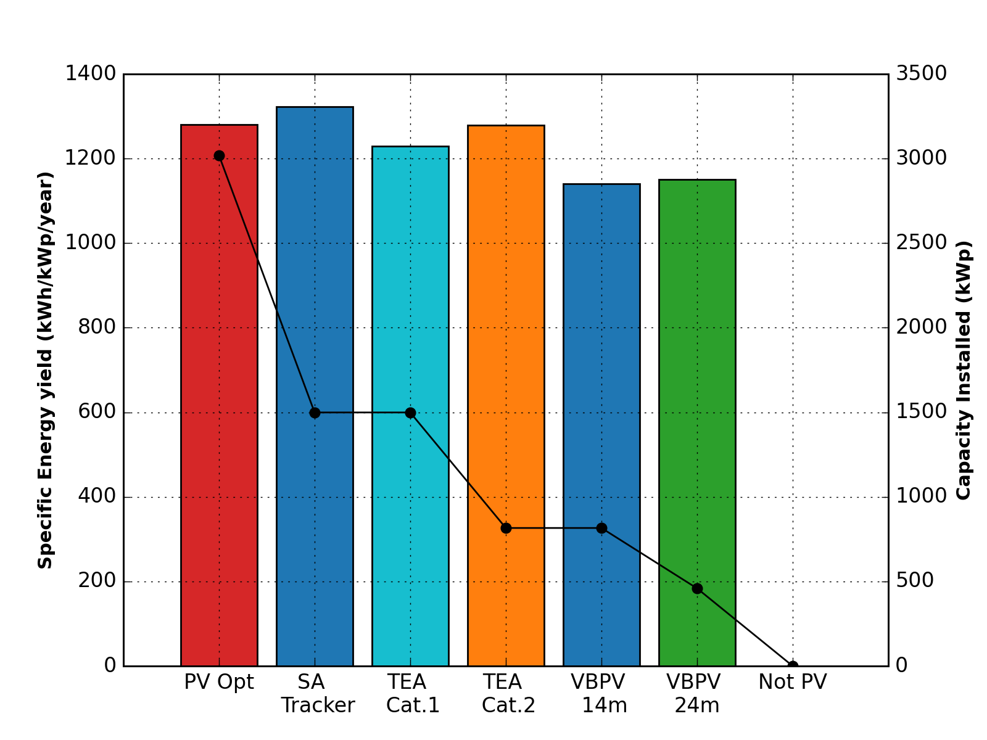
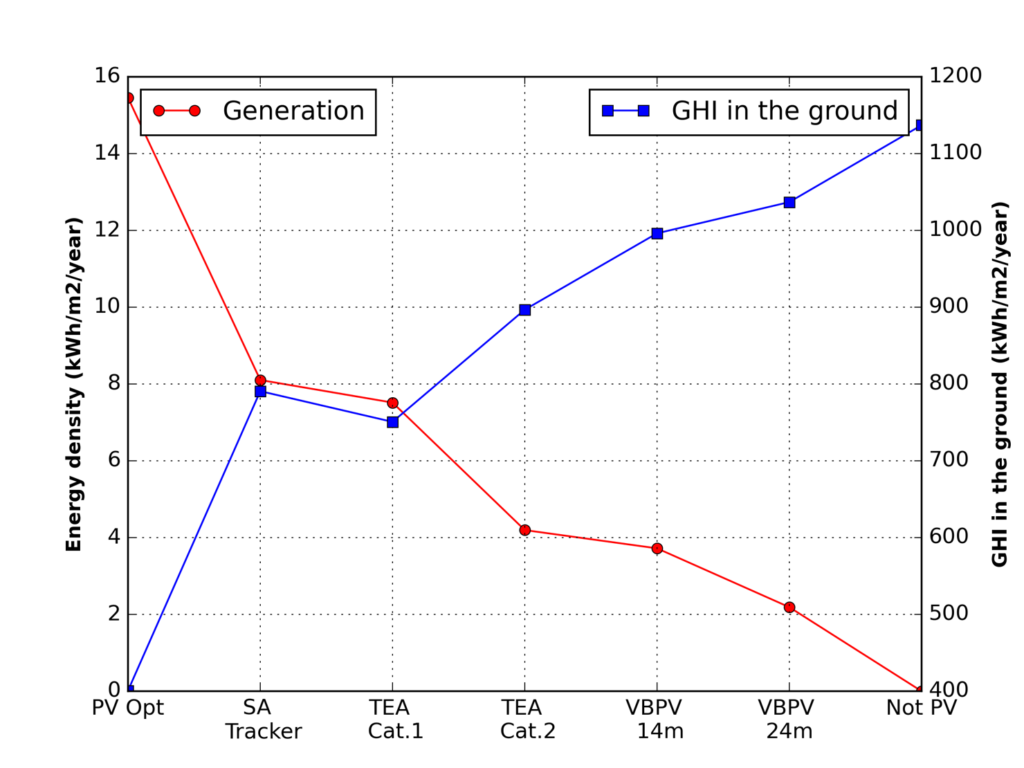
Light distribution is not the only factor that impacts on the crop. In another study [4], we developed an assessment performance for a vertical bifacial east-west system in the region of Chanco in Chile, a region with low precipitation. In this study, we found that a vertical system can produce around 10% less energy than a PV-optimised solar park, but the windbreak effect boosts the water savings for the field by up to 1,430m3/ha. Another interesting advantage of the vertical systems is the possibility to modify the generation profiles to achieve two peaks – one in the mid-morning and one in the mid-afternoon –generating a more flexible electricity generation profile compared to a typically oriented PV plant in which the midday generation peaks do not necessarily correspond with peak demand.
In pursuing further results, our research group built a demonstration site at our campus located in Bernburg, Germany. The demonstrator is helping us to test and analyse more variations and impacts with these systems. Also, the project goes one step further and explores the potential to integrate a third component: biodiversity. This concept, called AgriPVplus, combines energy and crop production with the integration of native wildflowers species to promote biodiversity conservation with species such as pollinators.
The integration of this species promotes the inclusion of further conservation benefits into the field. Land use by humans in activities such as farming and electrical generation have an impact on existing ecosystems that is rarely compensated. In the 75kWp PV test installation, we are exploring several combinations of crops with a monitoring that allows us to collect data to validate and refine the models that we have been developing. In parallel, it creates a living university campus, serving as real example for knowledge transfer centre to students, farmers, decision makers and the general public.
Enhanced land productivity
It is clear there is a potential for the strategic combination of crops and PV. Operating these systems synergistically on the same land could significantly enhance land productivity. Typically, the metric used for measuring the productivity of land is the Land Equivalent Ratio (LER). LER refers to the ratio of yield achieved for PV and food generation compared to the value required to produce the same amount of both outputs in two separate areas. Preliminary studies demonstrate that APV systems can increase the LER from 20-80%. In this case, it is clear that the maximisation of land productivity diversifies the revenue streams for the farmers using the same energy source, the Sun.
Besides the previously mentioned advantages, there are more possible enhancements that the system could provide, such as water productivity (mentioned before), water use productivity, capture of CO2, protection against over-irradiance of the crops and the possibility of further sustainability measures in the field resulting from the integration of conservation measurements. Additionally, APV can provide electricity to powered electric farming equipment such as seeders, sprayers, harvesters and tractors in general, reducing even more the carbon footprint in the farming industry.
Agrivoltaics presents an exceptional opportunity to balance land use for sustainable energy and food production, addressing the challenges of climate change, land scarcity and resource optimisation. By harnessing solar energy for both electricity generation and agriculture, agrivoltaic systems offer the potential to increase land productivity and diversify revenue streams for farmers, ultimately supporting the broader goals of carbon neutrality.
Preliminary studies show promising results, with significant increases in Land Equivalent Ratio (LER), enhanced water productivity, improved crop protection and more efficient use of resources. These findings suggest that agrivoltaics could reshape agricultural and energy practices by transforming fields into dual-purpose spaces that not only meet but exceed the traditional productivity of segregated land uses.
Furthermore, by integrating elements of biodiversity—such as wildflowers to attract pollinators—systems like AgriPVPlus offer additional ecological benefits, potentially enhancing soil health and promoting conservation. This approach paves the way for a more resilient farming ecosystem capable of withstanding changing climates and reduced water availability. The adaptability of agrivoltaic configurations—whether through elevated, bifacial, or tracking solar systems—allows for tailored solutions that can meet the specific needs of diverse crops and local climates. The table is served: clean energy and food—a powerful combination, all from the same energy source, the Sun.
Author
Hugo Sánchez Ortiz is a PhD candidate at Anhalt University of Applied Sciences in Köthen, Germany. His research focuses on the characterisation of microclimates for agrivoltaics and biodiversity integration to better understand interactions between PV and crops. He has worked in PV research since 2014, specialising in energy modelling, integration and monitoring.
References
[1] A. Goetzberger and A. Zastrow. 1982, “On the Coexistence of Solar-Energy Conversion and Plant Cultivation,” International Journal of Solar Energy, vol. 1, no. 1, pp. 55–69, doi: 10.1080/01425918208909875.
[2] Deutsches Institut für Normung. 2021., Agri-Photovoltaik-Anlagen – DIN SPEC 91434:2021-05. Anforderungen an die landwirtschaftliche Hauptnutzung.
[3] H. Sánchez Ortiz, S. Dittmann, C. Meza, and R. Gottschalg, 2023. “Comparison of Different AgriPV Layouts in Terms of Photovoltaic Energy Yield Output,” 40th European Photovoltaic Solar Energy Conference and Exhibition, doi: 10.4229/EUPVSEC2023/4DO.2.2.
[4] R. Bruhwyler et al.2023, “Vertical agrivoltaics and its potential for electricity production and agricultural water demand: A case study in the area of Chanco, Chile,” Sustainable Energy Technologies and Assessments, vol. 60, p. 103425, doi: 10.1016/j.seta.2023.103425